A Unique Petrified Tree Fern from Northeast China

ABSTRACT
A unique petrified tree fern displaying distinct patterns was recently discovered in northeast China, attracting considerable interest. This study provides a comprehensive set of data for this material obtained through standard gemological testing, petrographic observation, scanning electron microscopy, energy-dispersive spectroscopy, electron probe microanalysis, and Raman spectroscopy. The petrified tree fern exhibits colors ranging from golden yellow to brown, with additional variations caused by iron oxides. Chalcedony is the primary mineral component, while the presence of metastable moganite gives chalcedony its fibrous nature, contributing to the delicate structure of the samples. The surface pattern originates from plant structures preserved during silicification, further confirming the samples belong to the extinct Cretaceous period tree fern Tempskya sp.
Petrified wood is the fossilized remains of ancient trees that have undergone a process occurring over millions of years (Nowak et al., 2005; Yoon and Kim, 2008; Kim et al., 2010). During this process, dissolved SiO2 infiltrates the organic matter of buried trees, causing the precipitation of various crystalline siliceous minerals along the wood fiber cells. As a consequence, silicified fossils with preserved woody structures are formed (Murata, 1940; Rößler, 2000; Mustoe, 2008; Yu, 2016). However, most petrified wood is not of gem quality, lacking the necessary beauty and luster (Saminpanya, 2015).
Since 2010, a unique tree fern fossil recovered from riverbeds and secondary placers in northeast China has captured the attention of botanists (Cheng and Liu, 2017; Yang et al., 2018). This unusual tree fern, Tempskya, is the only genus in the family Tempskyaceae and occurred only in the Cretaceous (box A). These petrified tree fern fossils are composed of polycrystalline quartz. With its distinct patterns, this material has drawn the interest of gem collectors in the area (Xu, 2021). Lapidary artists value specimens for their use in cabochons, beads, and carvings (figure 1). As local jewelers recognized the worth of this material, they began to incorporate it into souvenirs and polished collectible items, adding to the appreciation of petrified tree fern (Pakhomova et al., 2020).
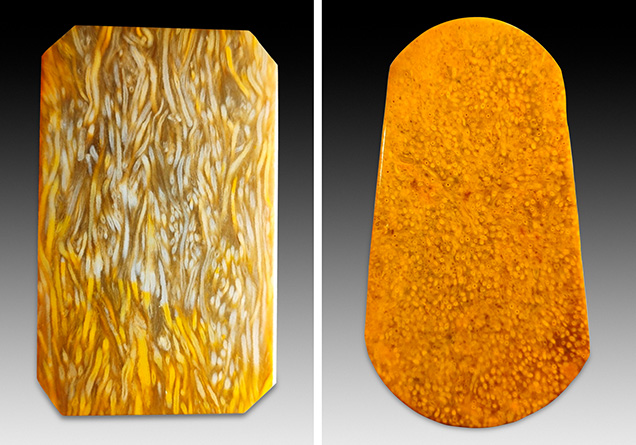

Petrified tree fern possesses desirable qualities as a gem material, characterized by its refined texture and preserved plant patterns (figure 2). Previous studies have focused primarily on paleogeography, paleoclimatology, and mineralogical aspects of petrified tree fern (Rößler, 2000; Witke et al., 2004), leaving its gemological significance largely unexplored, particularly with regard to microstructural analysis. The resemblance between petrified tree fern and tiger’s-eye (Holden, 2003) raises the need for accurate separation (figure 3). In view of these considerations, this study provides a comprehensive analysis of the microstructure and mineral composition of petrified tree fern.
MATERIALS AND METHODS
Six studied petrified tree fern samples (S1–S6) were procured from the gem market in Qiqihar, Heilongjiang Province, China. These specimens were originally collected by local villagers from fluvial deposits near the village of Henan, Keshan County, in Heilongjiang Province in the northern Songliao Basin (figure 4). The Songliao Basin is a Mesozoic-Cenozoic intracratonic basin across the Heilongjiang, Jilin, and Liaoning provinces of northeast China. One of the most prolific oil sedimentary basins in China, it is considered a long-lived terrestrial basin preserving a nearly complete Cretaceous sedimentary record (Yang et al., 2018). The Cretaceous strata in the basin are rich in biological fossils (Gao and Song, 1994; Kong et al., 2006). A discovery there in 2017 of a new species of tree fern fossil, Tempskya zhangii, has shed light on the paleogeographic environment of the Songliao Basin during the Cretaceous period (Yang et al., 2018).



The rough samples were rounded chunks covered with gray-white and brownish yellow weathering crust, indicating that the fossils had been transported by water over a long distance to their current sites (figure 5). The six samples were ground into polished slabs in the transverse direction (figure 6). Additionally, a sample of tiger’s-eye with an appearance similar to that of petrified tree fern was procured from the jewelry market in Wuhan for gemological and visual comparisons (figure 7).
The six petrified tree fern samples and the one tiger’s-eye sample were examined using standard gemological methods including visual observation, refractive index measurement, fluorescence reaction observation under long-wave (365 nm) and short-wave (254 nm) UV, and hydrostatic specific gravity testing.
The samples were prepared as thin sections both perpendicular and parallel to the plant texture direction, labeled as transverse and longitudinal, respectively. These sections were examined using an Olympus BX51 petrographic microscope. Scanning electron microscopy (SEM) was used to observe fractures in randomized directions of the petrified tree fern samples, performed by JEOL JSM-7800F. Working voltage and working distance were 15 kV and 10 mm, respectively. Energy-dispersive spectrometry (EDS) (Oxford X-Max 50) element mapping allowed the observation of chemical variation within the same regions.
Chemical composition was determined at the MNR Key Laboratory of Metallogeny and Mineral Assessment, Institute of Mineral Resources, Chinese Academy of Geological Science in Beijing. The analysis was performed with a JEOL JXA-8230 electron probe microanalyzer (EPMA) equipped with four wavelength-dispersive spectrometers. The sections were coated with an approximately 20 nm thin conductive carbon film prior to analysis. Accelerating voltage, beam current, and spot size were 15 kV, 20 nA, and 5 μm, respectively. Jadeite (silicon, aluminum), hematite (iron), rutile (titanium), potassium feldspar (potassium), and wollastonite (calcium) were used as standards. Data were corrected online using a modified ZAF correction procedure.
Raman spectra were recorded using a Horiba HR-Evolution Raman micro spectrometer with an argon-ion laser operating at 532 nm excitation between 1800 and 100 cm–1 and accumulating up to three scans.
RESULTS
Gemological Properties and Visual Appearance. The gemological properties of the six petrified tree fern samples are compared to the single tiger’s-eye sample in table 1. All of the samples displayed golden yellow to brown colors, but red impurities were observed in regions of samples S5 and S6. All petrified tree fern samples had a refractive index of 1.53–1.54 with specific gravity varying from 2.60 to 2.63. Their luminescence was inert under long-wave and short-wave UV radiation.
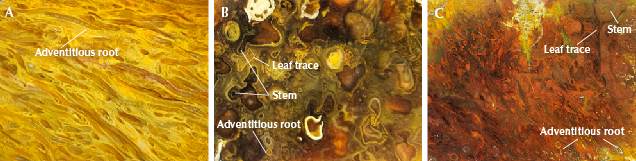
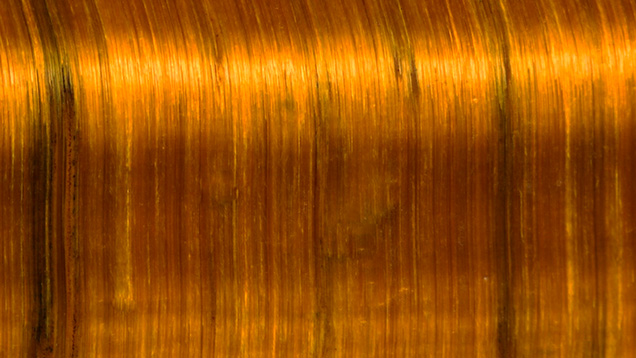
The polished surface of the petrified tree fern highlighted the internal structure of the plant, which differed from the parallel fiber structure found in tiger’s-eye. Three representative samples with distinctive internal textures of tree fern were selected for visual examination (figure 8). The unique surface patterns consisted of plant stems, adventitious roots, and leaf traces, appearing striped in a longitudinal section or irregularly rounded in a transverse section. The plant texture of sample S5 was entirely red, with tubular stems, dense adventitious roots, leaf bases, and a clear entanglement. Conversely, tiger’s-eye is characterized by lustrous golden brown bands that display banded chatoyancy (figure 9).
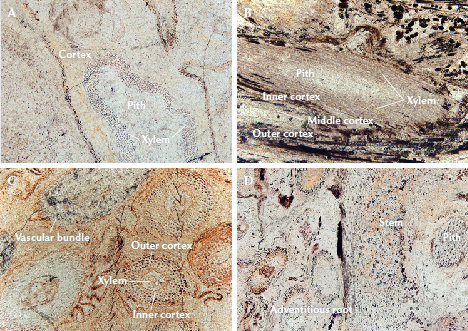
Microstructure. A polarizing microscope further revealed a well-preserved wood grain structure in the petrified tree fern samples. Individual stems were tubular and contained pith, vascular strands, xylem (the primary vascular tissue, which transports water and dissolved minerals from the roots to the leaves), and a cortex (figure 10A). The cortex was three-layered (figure 10B); the middle cortex had thick wall cells, while the outer and inner cortex were relatively thin. The pith was composed of thin wall cells that were elliptical in cross section. Each leaf trace had a single spindle-shaped vascular bundle (specialized tissues that run through and carry water and nutrition to different parts of the plant). The adventitious root was small in diameter, and the xylem was differentiated into two zones. The outer cortex consisted of large parenchyma cells. In contrast, the inner cortex was composed of thin wall cells (figure 10C). The solid, compact trunk was composed of stems embedded in a matrix of adventitious roots that were independent and intertwined with other roots (figure 10D).
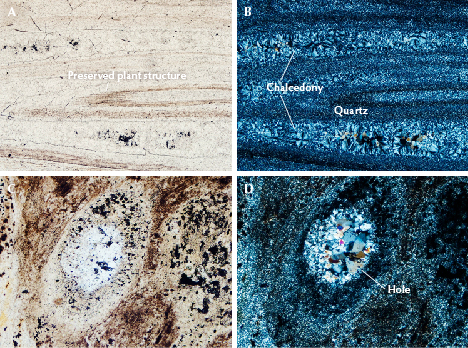
Mineralogically, the microcrystalline structure of petrified tree fern is diverse. Thin sections showed that samples were silicified by replacement, and chalcedony ± microcrystalline quartz made up more than 95% of their content. The quartz particles were rarely euhedral, mainly presenting as irregular microcrystals (less than 10 μm in diameter) and fibrous chalcedony spherulites (100–200 μm in diameter). The quartz particles were grayish white under plane-polarized light. According to Li (2016), wood fiber is the plant tissue component that is most resistant to decomposition and recrystallization, making it hard to mineralize. We therefore conclude that brownish yellow wood fiber structures are partly preserved in the petrified tree fern (figure 11A), but the decomposed part of the plant structure filled with chalcedony (figure 11B). In general, fossilization occurs when quartz has precipitated on the wall cells of samples, while chalcedony forms in open spaces such as vessels and veins (Hassan, 2017). As shown in figure 10B, fine-grained quartz filled in areas where woody structures were retained in the plant stems and roots. Conversely, radiating fibrous chalcedony crystallized in veins. Moreover, the subhedral quartz crystals were present in hollow structures such as vessels, veins, and holes (figure 11, C and D), while fine-grained quartz surrounded the hole.

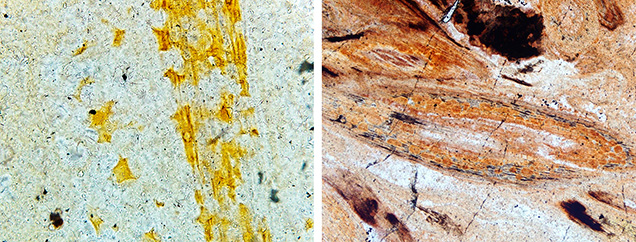
In addition, the plant structure was more evident near the shell of the petrified tree fern (figure 12) due to surface weathering. This weathering leads to fine crystalline decomposition and recrystallization of silica along the woody texture, reducing transparency and enhancing the distinct wood structure. The secondary regolith of the samples exhibited a wide distribution of yellow and red minerals that are associated with the woody structure (figure 13). However, the coloration is mainly affected by various minerals present during the weathering process.

SEM imaging revealed three distinct quartz textures in sample S4. The first texture consisted of cryptocrystalline quartz particles of 1 μm in diameter, grown in layers. The second was characterized by euhedral equant quartz crystals with particle diameters ranging from 1 to 5 μm (figure 14A), filling voids and partially visible due to a rhombohedral fracture surface. The first and second textures were both granular structures with low transparency. Conversely, the third texture contained semitranslucent regions composed of chalcedony fibers measuring approximately 5–10 μm in length. Figure 14B illustrates the structural characteristics of fibrous chalcedony. Most of the silica particles in the samples consisted of fine-grained quartz and chalcedony, with euhedral particles being less common.
The occurrence of vascular bundle structures on certain fracture surfaces mainly indicated atypical growth textures. Dense, oriented fibrous chalcedony formed around the pore wall. In the cross section of sample S4, a radial arrangement of fibers was evident (figure 14C). The vascular bundle structure showed a parallel arrangement in a longitudinal section (figure 14D). The agate-like geode growth structure suggests a silicification sequence from the outside (Scurfield and Segnit, 1984). After the SiO2 solution or colloid enters the vascular bundle cell or duct, quartz crystals grow in an oriented pattern around the cell wall. Crystal particles become progressively larger toward the center, displaying well-defined faces, which suggests that quartz had space for growth during formation, and crystals appeared to develop inward from the exterior.
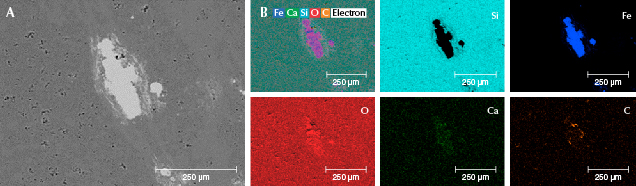
Element Mapping and Chemical Composition. In a backscattered electron image, a long columnar-shaped impurity in sample S6 can be seen in stark contrast to the host material (figure 15A). Element mapping revealed that the petrified tree fern matrix mainly contained silicon and oxygen, while the impurity minerals primarily consisted of iron and oxygen (figure 15B). Iron in the form of hematite is most likely the cause of color. At the same time, the boundary between the impurity minerals and the matrix contained a small amount of carbon corresponding to the residual carbonaceous matter in petrified wood (Li, 2016).
The backscattered electron image of the petrified tree fern shows a smooth surface. Five random spots on representative sample S1 were analyzed by EPMA. The results in table 2 agree well with the element mapping data, mainly containing SiO2 (97.94–99.43 wt.%) and a few impurity elements. This confirms that there is no clear difference in the position and composition of distinct plant textures in petrified tree fern.
Raman Spectroscopy. Raman spectroscopy was used to detect other phases that might be present in the samples (Kingma and Hemley, 1994). Figure 16 reveals that the Raman spectrum of petrified tree fern sample S2 is characterized by quartz mixed with moganite, indicated by the peak at 503 cm–1 (Heaney and Post, 1992; Hardgrove and Rogers, 2013). The Raman shifts present in petrified tree fern and tiger’s-eye occur predominantly at 127, 209, 262, 357, 402, and 465 cm–1, corresponding to quartz. Specifically, the scattering peak at 465 cm–1 is sharp and strong, related to the typical Si–O bending vibration of quartzite. Two peaks near 127 and 209 cm–1 correspond to the rotational or translational vibration of SiO4. Absorptions with very weak intensity at 262, 357, and 402 cm–1 are characteristic Raman scattering peaks for quartz.
DISCUSSION
The petrified tree fern examined in this study displayed golden yellow to brown colors, with color variations attributed to the presence of iron oxides, particularly hematite. Most samples were translucent to opaque, with deeper tones. Figure 12 shows the color-banded specimens resulting from the absorption of iron-bearing solutions along the wood grain. This observation suggests that the wood retains a degree of permeability (Mustoe and Acosta, 2016). Furthermore, the red and yellow exterior zones in the samples and the element mapping of iron oxide impurity minerals provide evidence that ferric oxide infiltrated the wood during the later stages of diagenesis (Saminpanya, 2016). Weathering during this process caused decomposition and subsequent recrystallization of cryptocrystalline quartz along the wood grain, reducing transparency and making the woody structure more visible (Stein, 1982; Li, 2016).
The unique characteristics of the six samples analyzed in this study, including the three-layered cortex, distinctive pith composition, the single fusiform vascular bundle, and the presence of stems embedded within an adventitious root matrix, indicated a connection to the Cretaceous tree fern Tempskya sp. (Clifford and Dettmann, 2005). Tree ferns are large, woody ferns that resemble trees and have trunk-like structures with fronds. The starting material of a tree fern, rather than a true tree, represents a notable variation within ordinary petrified wood.

Petrified tree fern and tiger’s-eye, both being forms of quartz, bear notable similarities in appearance and physical properties. Standard gemological properties are insufficient to distinguish between them, which has led to some confusion within the jewelry market. When examined in cross section, the adventitious roots of petrified tree fern exhibited a distinct oval pattern (figure 17A), while tiger’s-eye displayed a mosaic of fibrous bundles on end (figure 17B). These two patterns are very similar when viewed with the unaided eye.
In tiger’s-eye, crocidolite and columnar quartz grow simultaneously from opposing crack walls toward the center of the vein during a crack-seal process. The metasomatism only occurs when oxidizing fluids break down the crocidolite to hematite and goethite (Heaney and Fisher, 2003). Fibers of crocidolite are encased within columnar quartz and when grown parallel to each other (figure 17D) (Hu and Heaney, 2010). The formation of petrified wood, on the other hand, occurs under highly specific conditions (Pakhomova et al., 2020). Early research suggested that the petrification process is often associated with volcanic activity (Murata, 1940). Fallen or still upright trees become buried by volcaniclastics due to intense geological activity (Flörke et al., 1982). Ballhaus et al. (2012) observed that trees exhibit a clear propensity to absorb silica from hydrothermal fluids. Over time, the minerals crystallize, preserving the original structure and morphology of the organism in a fossilized form. The primary source of silica in this process is derived from ash silts (Saminpanya, 2015). Silica is considered the most active material for petrifying wood (Scurfield and Segnit, 1984). Through this process, petrified tree fern can preserve intricate patterns of fern fronds and the overall structure of the tree fern with remarkable detail (figure 17C). Therefore, the distinct silicification processes of petrified tree fern and tiger’s-eye result in unique structures and surface patterns, enabling their differentiation through careful macroscopic observation.
Buurman (1972) categorized the mineralization of wood into four processes: silicification, carbonation, phosphate accumulation, and sulfide accumulation. Among these, silicification involving quartz minerals is the most prevalent. Petrified tree fern has a chemical composition of SiO2, and there was no significant difference in chemical composition observed among the six different samples from this study, indicating that the specimens were thoroughly silicified. Quartz plays a crucial role in preserving the cell walls, resulting in the predominantly microcrystalline quartz composition of the preserved plant structures (Dietrich et al., 2013). Cell lumina, on the other hand, are filled with cryptocrystalline silica, specifically fibrous chalcedony, occupying the areas where plant structures have disappeared. Previous studies have confirmed that the fibrous character of chalcedony may arise from various forms of silica, including moganite as a metastable phase often found in fibrous chalcedony (Hassan, 2017; Mustoe, 2023). Raman spectroscopy was used to provide further evidence of constituent phases, enabling clearer identification of moganite in petrified tree fern (Heaney and Post, 1992; Götze et al., 1998). Furthermore, the Raman spectrum of tiger’s-eye lacks the signal of moganite, which might be a way to distinguish petrified tree fern from tiger’s-eye.

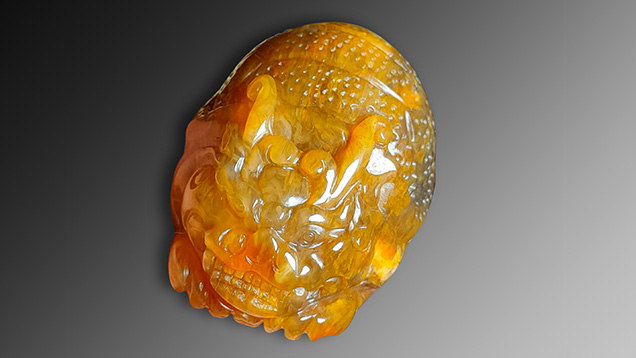
Additionally, highly crystalline quartz exhibits a hexagonal columnar growth (figure 18). An essential preservation characteristic is the presence of open vessels that provide space for the formation of well-developed quartz after the surrounding tissue has been silicified. The quartz filling this open space results from a separate mineralization event that occurred after dissolved silica levels dropped (Mustoe, 2023). However, the large particle size and rough edges of crystalline quartz in petrified wood cause light to be reflected and refracted internally, resulting in limited transparency (Lei, 2010). Conversely, fibrous chalcedony, the primary mineral commonly found in petrified tree fern, contributes to a delicate structure and enhances transparency (Miladinović et al., 2016; Lei, 2022). Therefore, the plant pattern filled with crystalline quartz can be more clearly distinguished from the surrounding chalcedony portions. In China, chalcedony petrified tree fern is highly valued as a lapidary material and ornamental stone, with high-quality specimens sought for the creation of carvings (figure 19).
CONCLUSIONS
Petrified tree fern from northeast China exhibited colors ranging from golden yellow to brown, with additional variations attributed to the presence of iron oxides. These specimens possessed distinct plant structures such as stems, adventitious roots, and leaf traces, resulting in a unique appearance. Although standard gemological testing proved insufficient in distinguishing petrified tree fern from tiger’s-eye, differences could be observed in their surface patterns, chatoyancy, and Raman spectra.
SEM-EDS and EPMA analyses confirmed the thorough silicification of the petrified tree fern samples, revealing a predominant SiO2 composition across various plant structures. Additionally, SEM and Raman spectroscopy identified chalcedony as the primary mineral component in petrified tree fern. The fibrous texture of chalcedony is induced by the presence of metastable moganite, contributing to the delicate structure observed. This material is remarkable for its beautiful pattern, representing an intriguing fusion of botany and gemology.